Inclusive Design: How Your Hands Don’t Work
Dan Formosa, Ph.D.
My friend JJ bought a new guitar. Playing it is causing some unexpected hand pain. The new guitar is not very different from his other one. He’s blaming the new guitar’s neck shape and how his hand fits around it. But that difference is subtle at best. Or maybe it’s the string gauge and scale length of the neck—both affect how tightly the strings need to be adjusted to be in tune. But again, I don’t think so—the differences between the guitars may be noticeable but would not be that significant.
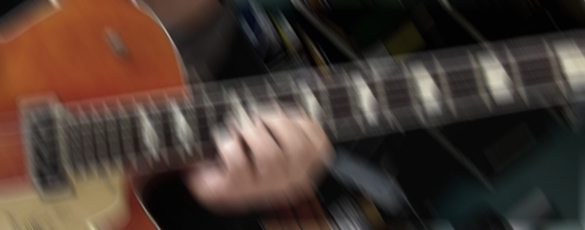
My advice is that he should look not just at his fingers, but also at his entire body posture. Study the possible effects of the new guitar’s larger size–its balance, the angle at which it’s being held, his shoulder, elbow and wrist angles—the whole arm. In understanding the hand you need to understand what’s happening at least up to the shoulder.
Musicians have company. Hand problems, including tendonitis and carpal tunnel syndrome, are common. Construction workers, hair stylists, people in the meat-packing industry, sewing machine operators, people who type a lot – all can have similar issues that can devastatingly impair the ability to perform. A combination of rapid finger movements, excessive hours of practice and sometimes-awkward hand positions can cause biomechanical havoc.
Design makes a difference. Women are more at risk than men. Obesity can be a contributing factor. Cold weather doesn’t help.
A look inside your hand
A hand’s mechanical issues can be many. Each hand contains 27 bones, 123 ligaments and 30 arteries. Movements are controlled by 17 small muscles within the hand itself, and 18 in the forearm. As a mechanism, it’s versatile. We use our hands to sense things—fingertips are sensitive to touch, able to distinguish minute qualities of an object such as subtle differences in shape and texture. Hands can sense cold and heat, airflow, dryness and moisture. Hands can accomplish tasks that require brute force, and others that require speed and precision. They can capably wield a heavy hand tool or help push a broken-down vehicle to the side of the road—but they can also thread a needle or play a violin. The hand is a stunning example of mechanical engineering.
It’s also complex. And like any complex mechanism, it’s easily susceptible to damage. Among its 27 bones are some of the smallest in the body. You’ve got smaller bones in the ear, but those are protected by your head. Your little toe is tiny and at risk of being stubbed, but shoes prevent that from occurring very often. Hands are generally exposed, often overworked and frequently at risk of some type of accident or mechanical failure.
Most of the muscles that enable your fingers to work—grabbing, pinching or squeezing—are not in your hand. Fingers are controlled by large muscles located in your forearm, one body part away. A well-orchestrated array of muscles, tendons and ligaments allows that to happen. Tendons are the string-like tissue that connect muscle to bone. Ligaments are similar in composition, but they connect one bone to another. Both are made of collagen and are surprisingly strong—lots of tensile strength. If you’ve ever had one tear somewhere on your body, you are probably well aware of their function and limitations.
Cuts, scrapes and burns can put your hand out of commission. An accident, some sort of physical trauma, can do damage also. The causes of these are obvious. Let’s focus on the more hidden risks, the problems caused by tasks a person is deliberately performing when using a product—problems that can be prevented by design. This understanding will also be used to improve performance because the same principles that reduce risk can optimize the ability of the hand to accomplish tasks that are quick or complex, or that require strength and endurance.
All that lifting, pushing, pulling, pressing, pinching, hanging, turning, luggage carrying, writing, typing, texting, mouse-clicking, scissoring, hammering, piano playing, rock climbing and fall-preventing, among other things, can take a toll. Improper wrist angles can exacerbate the detrimental effects of these behaviors. So can factors like temperature, vibrations and rapid repetitions of movement. The hand works like any other mechanical object, subject to the same laws of physics. When designing, it helps to consider the hand as a mechanism. Here’s a quick overview of how your hand works – and how it doesn’t.
Basics in Physiology
Muscles contract. That’s basically all they know how to do. Have your brain send your muscle a signal to fire and that muscle will get shorter. To expand a muscle you need to send a signal to an opposing muscle, stretching the first muscle back into place. That, or you need to rely on gravity or some other external force.
Consider what happens at your wrist. Grabbing something requires contraction of flexor muscles located in the underside of your forearm. Releasing your grip relies on the contraction of the extensor muscles located on the upper side of your forearm. Muscles are therefore either pulling from one side of the wrist or the other. The tendons that connect to those muscles run from your forearm to the tips of your fingers. Finger movement is complicated by the fact that those tendons need to pass through your wrist—and your wrist can bend.
Your wrist contains a series of eight small interlocking carpal bones. They allow your hand to swing side to side—the movement we use to wave down a New York City taxi. It can also flex forward and extend back, with approximately a 160-degree range of motion, although actual range will vary depending on the person. The carpal bones form a concave shape. As you look at your wrist with you palm facing you, stretching across the concave opening is a transverse, ribbon-like ligament. That combination of bones and ligament form the carpal tunnel through which the tendons must pass. Flexing a finger and then extending it causes the tendon to move back and forth through the tunnel.
With the wrist unbent, the tendons can pass through the tunnel easily. When the wrist is bent, the tendons pull against the transverse ligament. Parts start to rub against each other. Your grip will be much weaker. Rapid movement or excessive force coupled with that rubbing will cause tendons to become inflamed. The median nerve, sharing space within the carpal tunnel, can get squeezed, cutting off the blood supply. Repeated movements just make things worse. Common terms for the resulting problems, which occur often, include repetitive stress injury, cumulative trauma disorder, and carpal tunnel syndrome.
Then there are your fingers. Your index, middle, ring and little fingers each have three bones: distal (furthest from the wrist), middle and proximal. (For each, a fourth bone “hidden” in your palm extends from the wrist to the base of the finger.) Each finger therefore has three joints. The location of the furthest two joints can easily be seen by looking at the creases in the skin with your hand palm-side up. However, contrary to popular belief, the third joint is not located at the third crease in your finger—it’s in the center of your palm.
Like the transverse ligament in the wrist that forms the carpel tunnel, the finger joints have sheaths that keep the tendons in place. The three joints give each finger three places where things can go wrong. Repeated, rapid and excessive movements of the fingers too often or under too much stress will cause the tendons to inflame. A scroll wheel on a computer mouse, for example, can be one such cause. A small nodule can appear on the tendon, making it even less willing to pass through the sheath. A snapping, referred to as trigger finger, will be felt. The remedy calls for not bending that finger for a few weeks—usually achieved by using a splint.
Your middle finger is the strongest. Your index finger and ring finger are next strongest, and just about equal. The little finger, a.k.a. the pinkie, has less strength. Don’t write it off, however. For many tasks, such as squeezing a pair of pliers, the pinkie will also have the most leverage, since it’s furthest from the pivot point. It therefore contributes significantly.
Your thumb has two segments, distal and proximal, with a third in the palm. Muscles in the palm at the base of the thumb help it to close, open and flex. Through overuse—like excessive gaming or texting—its parts are similarly susceptible to breakdown.
Smaller Hands and the Triple Whammy
Designing to be inclusive means that products need to accommodate a wide range of sizes. Females can be at more risk for hand problems, partly because they are generally smaller than male hands, although sizes overlap. Smaller hands face three challenges when using products that don’t consider the lower portion of the size spectrum—a triple whammy:
• Smaller hands often mean smaller, less powerful muscles in the hand and forearm.
• A shorter grip span may necessitate operating or squeezing a device with fingertips, not the stronger middle segments of the fingers.
• The pinkie may not be able to reach around the object at all, and therefore can’t contribute.
Arthritis
Arthritis affects joints in the body. It can be very noticeable in the hands, significantly impairing function. It comes in several varieties, but the most common is osteoarthritis, a degeneration of the cartilage found at the end of bones. The result is pain, stiffness and weakness. Hand strength with arthritis can be one-third to one-quarter that of a healthy hand. More than 50 million adults in the US are afflicted with some form of arthritis, approximately one in four females and one in five males. It’s not just adults–more than 300,000 children in the US have arthritis.
For the US population, plotting an age curve for adults with arthritis results in a shape that’s more evenly distributed than you would expect. Designing products to accommodate someone with arthritis will help just about as many people below the age of 55 as above.
Sports and physical performance
Regardless of a person’s individual ability, designing to optimize performance and reduce injury requires a good understanding the mechanics of the hand–or for that matter the entire body. Products ranging from kitchen items to surgical tools need to be designed accordingly. Hardware and power tools need to anticipate a wide range of hand sizes, as well as the product’s weight and the forces being exerted. Sports equipment should enhance performance. Gloves and other items should be designed to work in harmony, freely allowing natural movements while protecting against unnatural movements and excessive impacts. Items that don’t fit, or that don’t take basic biomechanics into consideration will significantly degrade performance. Shape, weight, balance and choice of materials all play a role. And as with JJ’s guitar, it helps to take body positions and techniques into consideration.
Being Liked, Inclusively
If products are going to be usable and well liked, if they are being designed to perform with the body, and especially if they are to be considered inclusive, understanding basic mechanics of the body is a minimum requirement for a design team. It’s not quite right for designers or educators to say “we’re all about understanding people” when there is little consideration of, or lack of training in biomechanics. Mechanics of the hand serves as a good start, since the rest of the body behaves, and can be analyzed, in a similar way.
One other thing to know: given a complete biomechanical analysis, you would be surprised at the forces that need to be exerted by muscles, and the resultant forces acting on the bones, tendons and ligaments, when performing even simple day-to-day tasks. It can be magnitudes beyond the outward “visible” forces needed for the task. In studying those internal forces, and in view of the hand’s versatility and complexity, it’s not surprising that hand problems are common. We can save force analysis for a separate article.
– § –